Subscribe to the blog
Properties of WorkBeads 40 IEX resins: What makes them exceptional?
Apr 1, 2025 10:00:00 AM / The Bio-Works Team
All biomolecules used in therapeutic, diagnostic or other life science applications must be purified. Liquid chromatography is commonly used as it is fast, robust and scalable. The challenge in achieving a good purification process largely lies in selecting a resin with properties optimal for the target molecule of interest. The agarosebased WorkBeads™ resins described here have been shown to generate final products with very high purities and yields as well as exhibiting high loading capacities for a large number of biomolecules, especially for proteins, peptides and oligonucleotides. What follows is an in-depth explanation of how the bead properties generate such outcomes, due to their size, pore size, pore size distribution and their mechanical stability.
Chromatography agarose resins
Agarose-based chromatography resins consisting of highly cross-linked porous beads have been used for decades to purify and separate a diverse set of macromolecules. Agarose does not adsorb biomolecules non-specifically to any significant extent, it has good flow properties, and it can tolerate extremes of pH and ionic strength, i.e. it is compatible with harsh cleaning and regeneration solutions. For these reasons it is widely used in the separation and purification of biomolecules, at both laboratory and production scale.
There are however other types of resins and there has been an increase in the available range over the last decade. Agarose resins and methacrylic resins differ in their chemical composition, agarose being made of a natural polymer obtained from agar, while methacrylic resins are constructed/ developed from synthetic monomers. The second main difference has to do with molecular access to the resins. The internal porosity of agarose resins is often much larger than methacrylic resins and allows infiltration of very large biomolecules such as monoclonal antibodies which are approximately 150 kDa, whereas the methacrylic resins allow greater access for biomolecules below 100 kDa, such as peptides, proteins and oligonucleotides. The accessibility for larger biomolecules is however not the case for WorkBeads, which have smaller pore sizes in combination with a narrower pore size distribution (PSD) than traditional agarose-based resins, thus making WorkBeads resins a better choice for working with smaller molecules.
WorkBeads resins
WorkBeads resins consist of highly cross-linked, rigid agarose beads that are developed for both research and production-scale chromatography. A variety of resins exist, where the base matrix is derived with ligands used in ion exchange chromatography (IEX), affinity chromatography (AC) such as protein A resins and immobilized metal affinity chromatography (IMAC). There is also a subset of size exclusion chromatographic (SEC) resins and activated resins for which you can derivatize the base matrix with a ligand of your own specifications and create a specificity of your own choice. In this application note, the properties of WorkBeads resins are described; everything from the bead size and its composition to the pore size and the consequences of such properties, see Figure 1.
WorkBeads resins are produced according to ISO 9001:2015, and Regulatory Support Files (RSF) are available to assist the process validation and submissions to regulatory authorities.
Figure 1. An illustration of some important parameters, such as bead size, pore size and cross-linking chemistry, affecting the bead properties.
Rigidity and stability
Chemical stability
Agarose resins are very flexible and elastic, which means they are relatively forgiving and easy to work with. Any potential deformation of beads will be reversible when re-equilibrated as long as the column packing has been carried out below the resin’s max pressure limit. The chemical stability of the beads is very high, and they tolerate pH extremes, 2–14. The resin can therefore be regenerated under harsh cleaning conditions, such as 0.15 M phosphoric acid and 1–2 M sodium hydroxide. It can furthermore withstand relatively high organic solvent concentrations, as well as all commonly used buffer systems. It is the ligands derivatized onto the beads that set the limits, and some are more sensitive, such as protein A.
Temperature stability
Some applications are best performed at elevated temperatures, e.g. oligonucleotide purifications where WorkBeads 40Q is an excellent resin to use.
WorkBeads base matrices, as well as the ion exchangers, WorkBeads 40S and WorkBeads 40Q, have excellent tolerance to elevated temperatures such as 60° C and 82° C without any loss in ionic capacity, selectivity or rigidity after extended incubations.
WorkBeads base matrix
- Tolerates harsh cleaning conditions – pH 2–14 (e.g. 1 M NaOH) – organic solvents
- Tolerates elevated temperatures
- Autoclavable
- Rigid due to shorter cross-linker
Autoclaving is important if sterilization is a requirement in the process. WorkBeads 40S, WorkBeads 40Q and WorkBeads 40 TREN were autoclaved in neutral buffers using a standard autoclave program (121° C at 1 bar for 20 minutes). There was no change in performance regarding rigidity and selectivity post autoclaving indicating an extreme tolerance against high temperatures.
Mechanical stability
The agarose beads are, as stated, spherical and elastic. In order that high flow rates and backpressures can be tolerated without any deformation of the packed resin, however, it is also important that the beads are highly rigid. The cross-linking between the polysaccharide chains in the agarose adds strength and rigidity to the matrix so the beads work well in a packed column. WorkBeads resins are manufactured using a proprietary method that results in an exceptional mechanical stability due to shorter cross-linker.
Bead size vs. pore size
The combination of bead sizes and pore sizes are two crucial parameters to consider for optimal purification results. The application and scale determine the bead size range and the target molecule sets the boundaries for the optimal pore sizes to use.
Bead sizes
There is an inverse correlation between bead size and backpressure. The larger the bead, the lower the backpressure, but at the expense of selectivity and resolution. That is, the smaller the bead, the better separation between species, resulting in higher purities, but this generates very high backpressures. Therefore, all analytical columns contain small beads (2–5 µm) for the most complete possible separation between all species. This however cannot be scaled-up. Figure 2 illustrates the correlation between resolution and backpressure. A compromise between backpressure and selectivity must be made when determining the bead size. But note that the negative selectivity effects caused by a larger bead can partially be mitigated by a narrow pore size distribution.
Figure 2. The correlation between resolution and backpressure for decreasing bead sizes Purity Purification step
The sweet spot of bead size is at 45 µm, where a good separation is reached at modest backpressure. Although, some applications require larger beads, for example blood cell purifications, 100–200 µmbeads should be used. The manufacturing method for WorkBeads resins not only results in high mechanical stability but also results in porous beads with a tight size distribution. Figure 3 shows measured bead sizes for WorkBeads 40S (e.g. for peptide purifications) and WorkBeads 40Q (e.g. for oligonucleotide purifications) and their size distribution.
Figure 3. Measured bead size distributions for WorkBeads 40Q (blue) and WorkBeads 40S (green).
The bead size distributions are relatively similar for the eight different resins we measured in this study; WorkBeads 40S, WorkBeads 40Q, Capto™ SP ImpRes (Cytiva), Capto Q ImpRes (Cytiva), Praesto™ Jetted SP35 (Purolite), Praesto Jetted Q35 (Purolite), POROS™ XS (Thermo Fisher) and Nuvia™ HR-S (Bio-Rad). The average bead sizes were 35 µm – 60 µm, with the distribution range of ~30% below average and ~100% above average independent of the manufacturing technique (emulsification vs. jetting) and resin composition (agarose vs. polymeric) (data not shown). This study additionally shows that we see no advantage in using jetting manufacturing for obtaining a tighter bead distribution.
Pore sizes and pore size distribution (PSD)
Traditionally agarose beads have been used for proteins, including monoclonal antibodies, and other larger biomolecules such as plasmids and viruses. These molecules require large pores, since the pore size must be in a significant excess compared to the molecular size to allow an available high pore volume. However, with large pores a very broad pore size distribution is usually obtained, negatively affecting the molecules’ diffusion in and out of the pores. This heterogenous diffusion will affect the selectivity, with substantial peak broadening, and a subsequent decrease in purity. An optimal scenario would be a pore size ten times greater than the molecule size with a completely homogenous or very narrow PSD. A very narrow PSD results in uniform diffusion and high selectivity giving high purities. An optimal pore size also affects the loading capacity since the target molecule will have a higher accessible surface area.
A common technique for pore size determinations is inverse size exclusion chromatography (iSEC) in which pre-defined sizes of dextrans are used and analyzed with a reflective index (RI) detector. This technique enables measurement of the pore sizes in the beads in their natural environment, i.e. in a wet and swollen format.
Most of the various WorkBeads resin’s derivatizations are based on the WorkBeads 40/1000 base matrix with exception of protein A resins which have larger pore sizes due to the sizes of the monoclonal antibodies (mAbs) it is used to purify. In Figure 4 and 5 the pore sizes and pore size distributions are shown for WorkBeads IEX resins based on the 40/1000 base matrix compared to other agarose based IEX resins. These are good examples that how the loading dynamic binding capacity (DBC) and the selectivity are reflected by the pore sizes and the PSD, highlighting the importance of choosing a resin with optimal pore sizes for your target molecule.
Correlation between the pore size and PSD
For optimal pore volume access:
- Large molecules, such as mAbs (> 10 nm) – large pore sizes
- Smaller molecules, such as peptides (2–3 nm) – small pore sizes
Dynamic binding capacity (DBC)
Figures 5 and 6 present pore size and PSD measurements along with the available interaction volumes of WorkBeads 40S and WorkBeads 40Q and how this is reflected in the DBC for peptides and oligonucleotides respectively. Capto SP ImpRes (Cytiva) and Capto Q ImpRes (Cytiva) are also measured as references.
In Figure 4 the cation exchange resins are compared. The blue trace represents WorkBeads 40S and has a smaller and much narrower and well-defined PSD compared to Capto SP ImpRes. This is reflected in the surface area per volume resin plot (Fig. 4B). There is an interception point at 10 nm, i.e. molecules with a hydrodynamic radius of less than 10 nm (20 nm diameter) have a larger available surface area on WorkBeads 40S, whereas for molecules larger than 10 nm in radius there is a benefit in using Capto SP ImpRes.
Since the typical size of a peptide is 2–3 nm in diameter and less than 1.5 nm in radius, there is a much higher available interaction area using the smaller-pore WorkBeads resin for peptide purifications. DBC measurements also confirm this, as seen in Figure 4C, where almost 50% higher loading capacities are achieved using WorkBeads 40S for purification of peptides of different lengths and charge.
Figure 4. The pore size and the PSD can be seen for WorkBeads 40S (blue) and Capto SP ImpRes (green). (A) Pore size distributions shown as frequency vs. hydrodynamic radius of pores in nm. (B) Surface area per volume resin at different pore sizes by radius. Typical peptide, BSA and IgG sizes are illustrated in the plot as well as the interception point, i.e. the cut-off value when there is a switch of resin with the highest available interaction area. (C) DBC measurement of 2 peptides, an acidic 45 aa peptide and a basic 9 aa peptide, at a residence time of 4 minutes.
Figure 5. The read-out of the pore size and the PSD is shown here for WorkBeads 40Q (red) and Capto Q ImpRes (grey). (A) Pore size distributions shown as frequency vs. hydrodynamic radius of pores in nm. (B). Surface area per volume resin at different pore sizes in radius. Typical peptide, and BSA sizes are illustrated in the plot as well as the interception point, i.e. the cut-off value when there is a switch of resin with the highest available interaction area. (C) DBC measurement of an RNA oligonucleotide at a residence time of 2.5 minutes.
A similar outcome is seen for WorkBeads 40Q compared to Capto Q ImpRes purifying a 45 nucleotide RNA oligonucleotide as the target molecule. See Figure 5, where the red traces and bars represent WorkBeads 40Q. This oligonucleotide is a bit larger than the peptides used, but if the pores are much larger than the target molecule, an increased loading capacity is expected.
WorkBeads 40S and WorkBeads 40Q – advantages
- Smaller pore sizes → higher available interaction area → higher binding capacities
- More narrow pore size distribution (PSD) → better mass transport → increased purity
Purity
The purity of the final product is dependent on the efficiency and selectivity. The purity increases as the peaks become narrower (efficiency) and more symmetrical, as well as there being as much separation (selectivity) between peaks as possible (Figure 6A). The efficiency depends on both the bead size and the PSD. As shown, the PSD is much narrower for WorkBeads IEX resins than it is for other resins with the same bead size (Figures 4–5). This property generates a good and uniform molecular diffusion in and out of the pores, resulting in homogenous elution of the different species as defined and well separated peaks ensuring a higher purity of the final product. Selectivity runs demonstrate this effect, see Figure 6B, in which a mixture of 4 proteins with different isoelectric points was loaded onto either WorkBeads 40S (blue) or onto Capto SP ImpRes (green). The peaks in the blue trace are well separated, whereas the green peaks are not all baseline separated.
Figure 6. Selectivity and efficiency. (A) Illustration of selectivity and efficiency parameters. (B) Overlay of selectivity chromatograms for a mixture of 4 proteins (Concanavalin A, α-chymotrypsinogen A, ribonuclease A, and lysozyme) with different isoelectric points. WorkBeads 40S (blue) vs. Capto SP ImpRes (green).
Furthermore, the increase in purity due to the more defined pore size distribution of WorkBeads resins can be seen in comparative studies analyzing yield vs. purities, see Table 1. Case studies 1 and 2 are peptide purifications where the yield is set, and these pools are analyzed for purities. The third case study is the outcome of an oligonucleotide purification in which both the yield and the purity were improved as compared to the other resin tested.
Table 1. Purity and yield data from different purification studies using different resins.
Conclusion
The aim of this application note is to give a detailed description of WorkBeads 40 resins, their properties and how these properties are reflected in the purification performance. Below is a summary.
WorkBeads 40 properties
- Agarose beads → tolerate harsh cleaning conditions, high temperatures and even autoclaving
- Tighter cross-linking → rigid beads → better flow properties
- 45 µm bead → balance between lab scale and production scale
- Narrow pore size distribution → higher selectivity and efficiency → higher purities
- Smaller pore sizes → higher available interaction area → higher DBC for molecules < 20 nm
Ordering information
Visit www.bio-works.com for information regarding all WorkBeads resins.
Orders: sales@bio-works.com or contact your local distributor.
For more information about local distributor and products visit www.bio-works.com or contact us at info@bio-works.com
bio-works.com
Bio-Works, WorkBeads and GoBio are trademarks of Bio-Works Technologies. All third-party trademarks are the property of their respective owners. © Bio-Works. All goods and services are sold subject to Bio-Works terms and conditions of sale. Contact your local Bio-Works representative for the most current information. Bio-Works, Virdings allé 18, 754 50 Uppsala, Sweden. For local office contact information, visit bio-works.com/contact. AN 40 300 011 BA
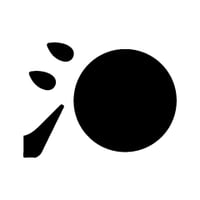