Subscribe to the blog
How diversity in peptide therapeutics can revolutionize medicine
October 17, 2024 / The Bio-Works Team
More than 80 peptide drugs have been approved by the FDA so far, and many more are in clinical development for a wide range of diseases. Peptide drugs have substantial therapeutic potential because of their high binding affinities, selectivity and specificity, and good efficacy. Their already proven market success and economic potential are also helping to drive many exciting approaches to overcome their shortcomings. We’ve summarized just a few of the current developments of peptide drugs that give reason to believe in an exciting future for these molecules.
Therapeutic peptides are one of the hottest topics in pharmaceutical research
Peptide drugs are a unique class of pharmaceutical agents with molecular weights of 500-5000 Da, and their development as drugs has become one of the hottest topics in pharmaceutical research.
The first peptides studied as therapeutics were natural extracted hormones such as insulin, oxytocin, vasopressin, and gonadotropin-releasing hormone (GnRH).
But then solid-phase peptide synthesis revolutionized the field in the 1950s and 1960s by providing quick and reliable access to nearly all peptides. Twenty years later, gene manipulation techniques began to enable the production of the first recombinant therapeutic peptides (e.g. insulin in 1982).
New peptide drugs for an even broader range of targets were identified when in vitro display methods (e.g. phage, mRNA) were developed and combinatorial synthetic libraries became more advanced. There has also been heavy research into strategies to improve the medicinal properties of peptides.
All these advances have boosted the peptide market to the extent that more than 80 peptide drugs have now been approved by the FDA, and many more are in clinical development. They cover a wide range of disease areas, such as cancer, endocrinology, and metabolic, cardiovascular, and bone diseases.
Several strategies are available for producing peptides as therapeutics. On the one hand, there is chemical or enzymatic synthesis, and on the other hand, expression systems, either cell-free or recombinant, in transgenic animals or in plants. This gives a wide range of possibilities for creativity and experimentation.
Peptide drugs hold enormous promise
There are significant advantages to using peptides as drugs. They generally have high binding affinities, high selectivity and specificity and good efficacy, due to their larger size and higher number of hydrogen-bond interactions.
Peptides can bind to surfaces, so they can be used to target ‘undruggable’ targets. A hot area of interest in peptide therapeutics currently is focused on macrocyclic peptides that have a unique size and high binding surface area, so they can address protein–protein interactions (PPI) and other undruggable targets.
Peptides usually have the potential to be safe drugs thanks to their high specificity and target affinity. They are broken down in the body into non-toxic amino acids or are filtered out by the kidney without significant CYP metabolism, reducing the risk for drug–drug interactions. In comparison to biologics (especially therapeutic proteins), peptides show lower immunogenicity.
Peptides have longer shelf-life than other biologics and good stability at room temperature; their lack of higher-order structure means they cannot denature.
The automatization of chemical synthesis makes peptide production straightforward since protocols are scalable. Chemical synthesis also allows chemical modifications and the creation of cyclic peptides and peptidomimetics.
There does exist some issues
Peptides have some shortcomings that cause problems with their use as medicines. To combat these, you can either change the chemical structure of the peptide or use drug formulations and delivery approaches that do not change the structure of the peptide.
One particular problem is the limited uptake of peptide drugs after oral administration.
A peptide can be chemically degraded in the acid of the stomach or be destroyed by enzymes (peptidases/proteases) in the GI tract and the gut wall that specifically chew up peptides.
The circulation half-life of peptides is also limited, mainly due to protease digestion and clearance by the kidneys. Proteases in blood plasma or enzymes in the target tissue can turn a peptide into useless metabolites.
Protease stability is, therefore, a big issue in the success of peptides as drugs, and there is a whole range of peptide modifications that address this.
- Proteases and peptidases work on a native free amino and carboxy terminus, so stability can be improved by modifying the N-terminus, for example, by acetylation. N-methylation also generates a peptide that is barely recognizable to peptidases.
- Proteases and peptidases work on linear peptide sequences, so turning the peptide chain into a closed loop has been a successful strategy to stabilize secondary structures such as α-helices and β-turns.
- Peptidases recognize L-amino acids, so exchanging these with the corresponding D-amino acids can stabilize the peptide. D-amino acids have been used for example in the development of somatostatin (L-Trp to D-Trp). N-alkylated (mostly N-methylated) amino acids have also been successfully used to increase proteolytic stability, for example in substance P, endothelin, neurotensin and many more.
- Unusual or non-canonical amino acids can increase the stability of therapeutic peptides, as they are not recognized by human proteases. These synthetic amino acids enable new applications for peptides. For example, they can incorporate reactive side chains for crosslinking.
- Strategies such as conjugation to polyethylene glycol or Fc domains improve peptide survival in the blood and also improve enzymatic stability of the conjugated peptides due to shielding effects.
With all these and other advances in peptide engineering, oral peptide drugs have now been developed, such as linaclotide - a peptide drug used to treat irritable bowel syndrome, and semaglutide - a peptide drug used to treat diabetes.
Peptides are strengthening other drugs
Maybe the most noteworthy examples of the potential of peptide therapeutics can be seen in combination with other molecules. They hold great promise of powerful therapeutic effects.
Peptides are being used to create targeted therapies that selectively affect specific molecules or cells in the body. For example, monoclonal antibodies that are joined to peptides can target cancer cells and deliver chemotherapy drugs specifically to the tumor, helping to avoid side effects in the rest of the body.
Peptides can improve effectiveness when combined with other drugs or therapies. For example, peptide-based vaccines can be combined with immune checkpoint inhibitors to enhance the immune response to cancer cells so the body can destroy the tumor itself. Another emerging approach involves the use of personalized peptide-based vaccines that attack neoantigens which are unique tumor-produced antigens not present anywhere else in the body. This creates a more focused and stronger anti-tumor effect.
In gene therapy, peptides are on the front line of the quest for new, non-viral vectors to avoid the immunogenicity and complicated manufacturing of viral vectors. Most recently, peptides are being studied as new promising biomaterials for gene delivery due to their desirable properties and biocompatibility. Peptide-based carriers can protect the gene therapeutic from destruction and aid its delivery to the target cells. Naturally-derived as well as man-made or hybrid peptides of various sizes and structures have been used for gene delivery.
Chemical modification and combination approaches have now grown to include many successful examples and methods, and we can see an enormous range of peptide variants currently in development. They can have vastly different physical and chemical properties, so it is no surprise that processes currently used for peptide manufacturing and production will quickly become stretched.
In particular, new peptide purification methods will need to be developed that can handle the increasing diversity of biologic drugs. Purified products are needed before pre-clinical studies are done and could be a bottleneck in assessing the potential of a new drug candidate. The future lies in flexibility and a high degree of expertise in developing purification solutions.
In the meantime, custom purification solutions are becoming more and more popular. Read more about the diversification of biopharmaceuticals and the customization of purification solutions here.
Technology breakthroughs guarantee long-term success
Although the promise of peptide drugs began with natural hormones, the discovery and development have now shifted all the way from copying the natural molecules to designing new peptides from scratch with desired biochemical and physiological properties. Major breakthroughs on many levels of molecular biology, peptide chemistry, and delivery technologies have brought the field to where it is today.
Considering their huge therapeutic potential, their already proven market success, and economic potential, we would expect therapeutic peptides to continue to attract scientific and investment interest and to continue to achieve long-term success.
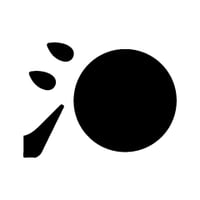